Stereolithography 3D printing for the investigation of acoustic focusing
I Made Miasa, Department of Mechanical and Industrial Engineering, Universitas Gadjah Mada, Indonesia
Adhika Widyaparaga, Department of Mechanical and Industrial Engineering, Universitas Gadjah Mada, Indonesia
Sucipto Sucipto, Department of Mechanical and Industrial Engineering, Universitas Gadjah Mada, Indonesia
Witnadi Dardjat Premiaji, Department of Mechanical and Industrial Engineering, Universitas Gadjah Mada, Indonesia
Abstract
In our environment, acoustic sound waves transform into undesirable noise when their intensity exceeds 100 dB, prompting a need for effective mitigation strategies. In recent years, there has been increasing interest in utilising sound/noise and acoustics for energy harvesting, especially for low-power electronic devices committed to clean renewable energy sources. Metamaterials, with a spotlight on metalens, are emerging as a promising solution for precise sound focusing and energy conservation. This study delves into the intricate process of fabricating metalens through Stereolithography (SLA) 3D printing, unravelling their acoustic focusing capabilities. Metalenses, equipped with intricately designed labyrinthine unit cells tailored for manipulating reflected wave phases, materialize through the precision of SLA 3D printing, forming a sophisticated multilateral structure. The experimental framework for acoustic focusing integrates essential components such as a waveguide, speaker array, metalens, acoustic foam, and a sound level meter. The resultant metalens, composed of 22-unit cells with diverse dimensions, distinctly demonstrate robust acoustic focusing capabilities. Calibration procedures are systematically applied to ensure uniformity of speaker array output and to create a carefully controlled acoustic environment. Sound level measurements clearly depict zones of mutually reinforcing resonance heights, while, conversely, there are also zones of mutually attenuating sound. The complex interplay of sound waves through the metalens, intricately guided by the design of the unit cells, decisively determines the degree of acoustic focus achieved. The SLA 3D printed metalens emerges as a compelling manifestation of effective sound concentration, poised for potential applications in the realm of acoustic energy harvesting. Nevertheless, the study's consequential findings beckon further scientific exploration, prompting an in-depth comprehension of the nuanced impacts of input frequency and potential heating phenomena.
Keywords
Full Text:
PDFReferences
J. Ivošević, T. Bucak, and P. Andraši, “Effects of interior aircraft noise on pilot performance,” Appl. Acoust., vol. 139, no. March, pp. 8–13, 2018, doi: 10.1016/j.apacoust.2018.04.006.
H. M. Noh, “Acoustic energy harvesting using piezoelectric generator for railway environmental noise,” Adv. Mech. Eng., vol. 10, no. 7, pp. 1–9, 2018, doi: 10.1177/1687814018785058.
A. Pascale et al., “Road traffic noise monitoring in a Smart City: Sensor and Model-Based approach,” Transp. Res. Part D Transp. Environ., vol. 125, no. June, p. 103979, 2023, doi: 10.1016/j.trd.2023.103979.
M. R. Monazzam et al., “Investigation of occupational noise annoyance in a wind turbine power plant,” J. Low Freq. Noise Vib. Act. Control, vol. 38, no. 2, pp. 798–807, 2019, doi: 10.1177/1461348418769162.
W. Yu-qin and D. Ze-wen, “Influence of blade number on flow-induced noise of centrifugal pump based on CFD/CA,” Vacuum, vol. 172, no. August 2019, p. 109058, 2020, doi: 10.1016/j.vacuum.2019.109058.
M. Yuan, Z. Cao, J. Luo, and X. Chou, “Recent developments of acoustic energy harvesting: A review,” Micromachines, vol. 10, no. 1, 2019, doi: 10.3390/mi10010048.
Y. Wang et al., “A renewable low-frequency acoustic energy harvesting noise barrier for high-speed railways using a Helmholtz resonator and a PVDF film,” Appl. Energy, vol. 230, no. July, pp. 52–61, 2018, doi: 10.1016/j.apenergy.2018.08.080.
C. Kim, J. Kim, and W. Jeon, “Realization of an acoustic metalens exhibiting broadband high transmission,” J. Sound Vib., vol. 529, Jul. 2022, doi: 10.1016/j.jsv.2022.116910.
S. Qi, Y. Li, and B. Assouar, “Acoustic Focusing and Energy Confinement Based on Multilateral Metasurfaces,” Phys. Rev. Appl., vol. 7, no. 5, May 2017, doi: 10.1103/PhysRevApplied.7.054006.
A. Spadoni and C. Daraio, “Generation and control of sound bullets with a nonlinear acoustic lens,” Proc. Natl. Acad. Sci. U. S. A., vol. 107, no. 16, pp. 7230–7234, Apr. 2010, doi: 10.1073/pnas.1001514107.
C. M. Donahue, P. W. J. Anzel, L. Bonanomi, T. A. Keller, and C. Daraio, “Experimental realization of a nonlinear acoustic lens with a tunable focus,” Appl. Phys. Lett., vol. 104, no. 1, Jan. 2014, doi: 10.1063/1.4857635.
G. do Gildean, E. F. Vergara, L. R. Barbosa, A. Lenzi, and R. S. Birch, “Sound absorption metasurface with symmetrical coiled spaces and micro slit of variable depth,” Appl. Acoust., vol. 183, p. 108312, 2021, doi: 10.1016/j.apacoust.2021.108312.
D. S. Nagaraju, R. L. Krupakaran, C. Sripadh, G. Nitin, and G. Joy Joseph Emmanuel, “Mechanical properties of 3D printed specimen using FDM (Fused deposition modelling) and SLA (Stereolithography) technologies,” Mater. Today Proc., no. September, 2023, doi: 10.1016/j.matpr.2023.09.223.
S. Wickramasinghe, T. Do, and P. Tran, “FDM-Based 3D printing of polymer and associated composite: A review on mechanical properties, defects and treatments,” Polymers (Basel)., vol. 12, no. 7, pp. 1–42, 2020, doi: 10.3390/polym12071529.
V. D. Sagias, K. I. Giannakopoulos, and C. Stergiou, “Mechanical properties of 3D printed polymer specimens,” Procedia Struct. Integr., vol. 10, pp. 85–90, 2018, doi: 10.1016/j.prostr.2018.09.013.
C. Curti, D. J. Kirby, and C. A. Russell, “Systematic screening of photopolymer resins for stereolithography (SLA) 3D printing of solid oral dosage forms: Investigation of formulation factors on printability outcomes,” Int. J. Pharm., vol. 653, no. January, p. 123862, 2024, doi: 10.1016/j.ijpharm.2024.123862.
M. Kurimoto, Y. Manabe, S. Mitsumoto, and Y. Suzuoki, “Layer interface effects on dielectric breakdown strength of 3D printed rubber insulator using stereolithography,” Addit. Manuf., vol. 46, no. March, p. 102069, 2021, doi: 10.1016/j.addma.2021.102069.
H. Bin Lee, M. J. Noh, E. J. Bae, W. S. Lee, and J. H. Kim, “Accuracy of zirconia crown manufactured using stereolithography and digital light processing,” J. Dent., vol. 141, no. January, p. 104834, 2024, doi: 10.1016/j.jdent.2024.104834.
DOI: https://doi.org/10.21831/jeatech.v5i1.71672
Refbacks
- There are currently no refbacks.
Copyright (c) 2024 Journal of Engineering and Applied Technology

This work is licensed under a Creative Commons Attribution 4.0 International License.
Our journal has been indexed by:

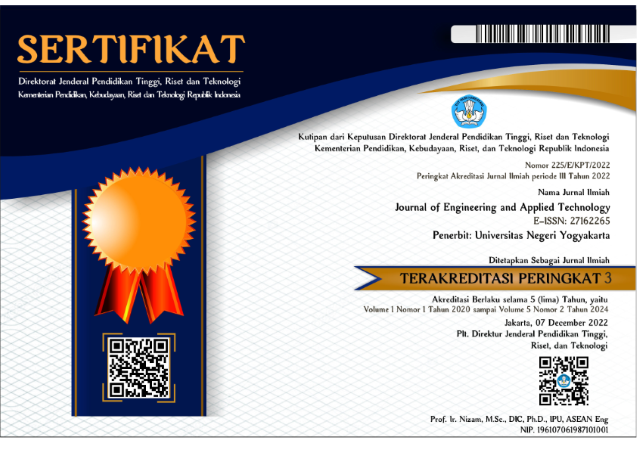
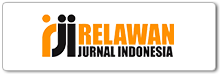
Journal of Engineering and Applied Technology (JEATech) by Faculty of Engineering UNY is licensed under a Creative Commons Attribution-ShareAlike 4.0 International License.